Bioreactor for Induced Pluripotent Stem Cell: Unleashing the Potential of Regenerative Medicine
In the rapidly evolving field of regenerative medicine, induced pluripotent stem cells (iPSCs) have emerged as a revolutionary source of cells with the potential to transform therapeutic approaches for a wide range of diseases and conditions. Central to the successful cultivation and manipulation of iPSCs is the development and utilization of specialized bioreactors.
This article aims to provide a comprehensive exploration of bioreactors designed for iPSCs, covering their design principles, functionality, applications, and the challenges and opportunities they present.
The design of a Cell Tainer bioreactor for iPSCs is a complex and multi-faceted undertaking that requires a deep understanding of both stem cell biology and engineering principles. The primary objective is to create an environment that closely mimics the physiological conditions necessary for the maintenance and proliferation of iPSCs while allowing for precise control and manipulation of the culture parameters.
One of the key considerations in the design is the selection of materials that are biocompatible and do not induce any adverse effects on the cells. The bioreactor vessel itself is often fabricated from materials such as polymers or glass that can withstand sterilization processes and provide a stable surface for cell attachment and growth.
The internal architecture of the bioreactor is carefully engineered to ensure efficient mass transfer of nutrients, gases, and metabolites. This typically involves the incorporation of agitation or perfusion systems to ensure uniform distribution of substances throughout the culture volume. Additionally, the design may include features such as microfluidic channels or scaffolds to provide structural support and enhance cell-cell interactions.
Temperature and pH control systems are integral components of the bioreactor to maintain the optimal conditions for iPSC survival and proliferation. Sensors are strategically placed to monitor these parameters in real-time and trigger corrective actions when necessary.
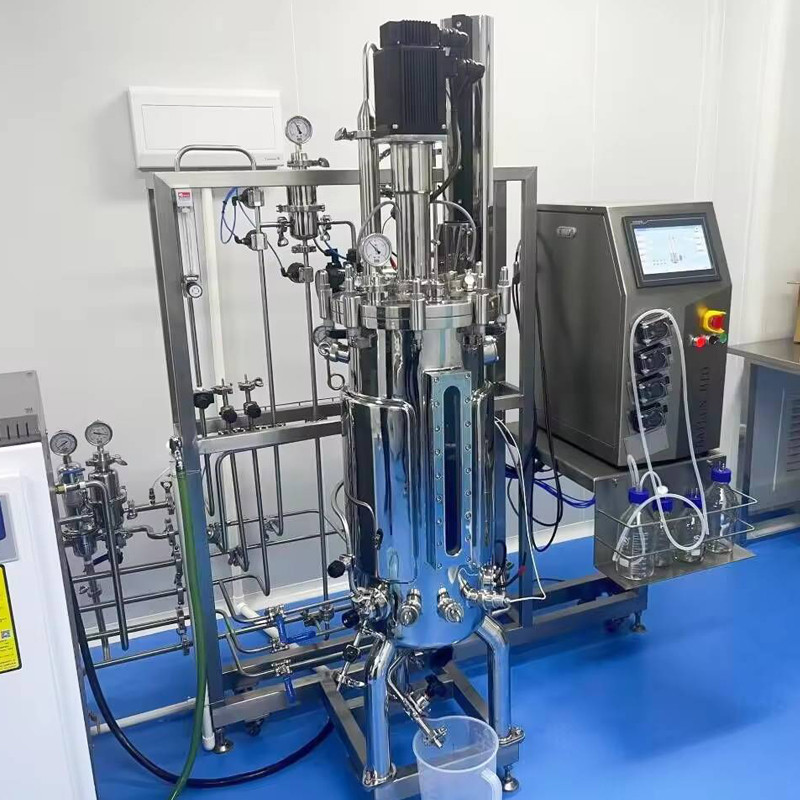
The functionality of a bioreactor for iPSCs goes beyond simply providing a physical container for cell growth. It is equipped with advanced control systems that allow for precise regulation of various environmental factors. For example, the concentration of growth factors, cytokines, and small molecules can be precisely controlled to direct the differentiation of iPSCs into specific cell lineages.
The bioreactor also enables continuous monitoring of cell health and function. Non-invasive techniques such as optical imaging or electrochemical sensing can be employed to assess parameters such as cell viability, proliferation rate, and the expression of specific markers. This real-time feedback is crucial for making timely adjustments to the culture conditions and ensuring the quality and homogeneity of the iPSC population.
One of the significant applications of iPSC Bio Fermenter bioreactors is in the field of drug discovery and development. iPSCs can be differentiated into various cell types relevant to disease, such as cardiomyocytes for cardiac disorders or neurons for neurological conditions. These cells can then be exposed to potential drug candidates within the bioreactor, allowing for the assessment of drug efficacy and toxicity in a physiologically relevant context.
In regenerative medicine, the bioreactor plays a crucial role in generating large quantities of functional cells for transplantation. By optimizing the culture conditions, iPSCs can be efficiently differentiated into therapeutically relevant cell types, such as pancreatic beta cells for diabetes or hepatocytes for liver diseases.
The controlled environment of the bioreactor helps ensure the cells have the appropriate characteristics and functionality before being transplanted into patients.
Bioreactors for iPSCs also have implications in the study of disease mechanisms.
By recapitulating the disease environment within the bioreactor and using iPSCs derived from patients with specific genetic disorders, researchers can gain insights into the underlying cellular and molecular processes that contribute to the disease progression.
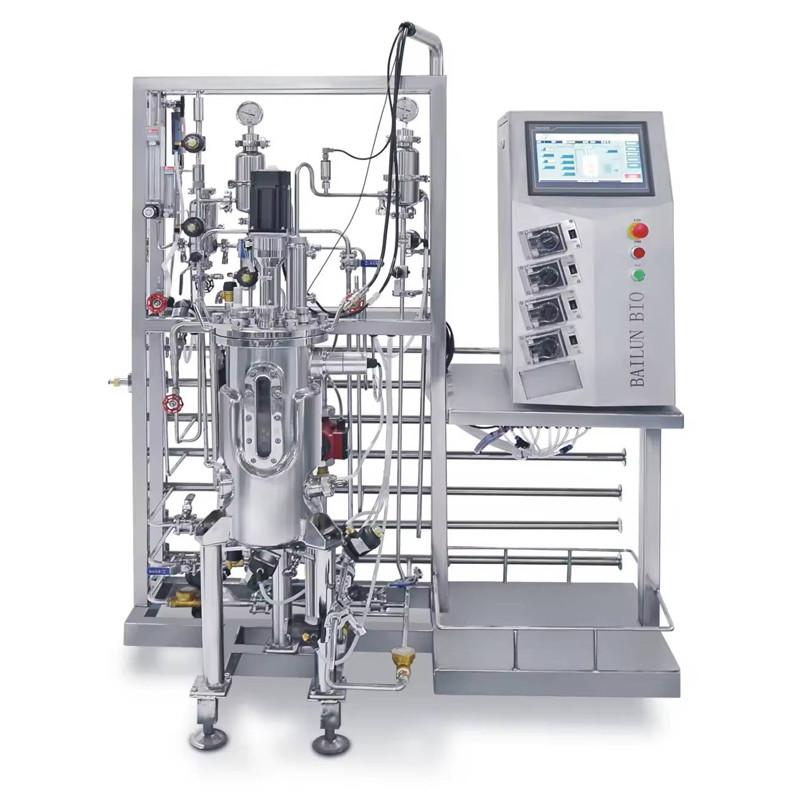
However, the use of iPSC bioreactors is not without challenges. One of the primary concerns is maintaining the genomic stability and epigenetic integrity of iPSCs during prolonged culture in the bioreactor. Changes in the genetic or epigenetic landscape can potentially affect the functionality and safety of the derived cells.
The scalability of the bioreactor systems is another critical issue. While small-scale bioreactors are valuable for research purposes, the translation to large-scale production for clinical applications requires the development of efficient and cost-effective strategies.
Furthermore, the regulatory and ethical considerations associated with the use of iPSCs and their derivatives in bioreactor systems are complex and need to be navigated carefully to ensure the safety and efficacy of any therapeutic products.
Despite these challenges, the potential of bioreactors for iPSCs is immense.
Ongoing research and technological advancements are focused on addressing these limitations and maximizing the benefits of this technology. The integration of novel materials, improved sensing techniques, and advanced control algorithms holds the promise of more sophisticated and efficient bioreactor systems.
In conclusion, the development of bioreactors specifically tailored for induced pluripotent stem cells represents a significant step forward in the field of regenerative medicine. By providing a controlled and scalable environment for the growth and manipulation of iPSCs, these bioreactors have the potential to unlock new therapeutic possibilities and contribute to the advancement of personalized medicine.
Continued innovation and collaborative efforts between scientists, engineers, and clinicians will be crucial in realizing the full potential of this exciting technology.